We redesigned the
4 MILLION
base pair E. coli genome and ordered it from a factory in 400 pieces of DNA, produced by chemical synthesis
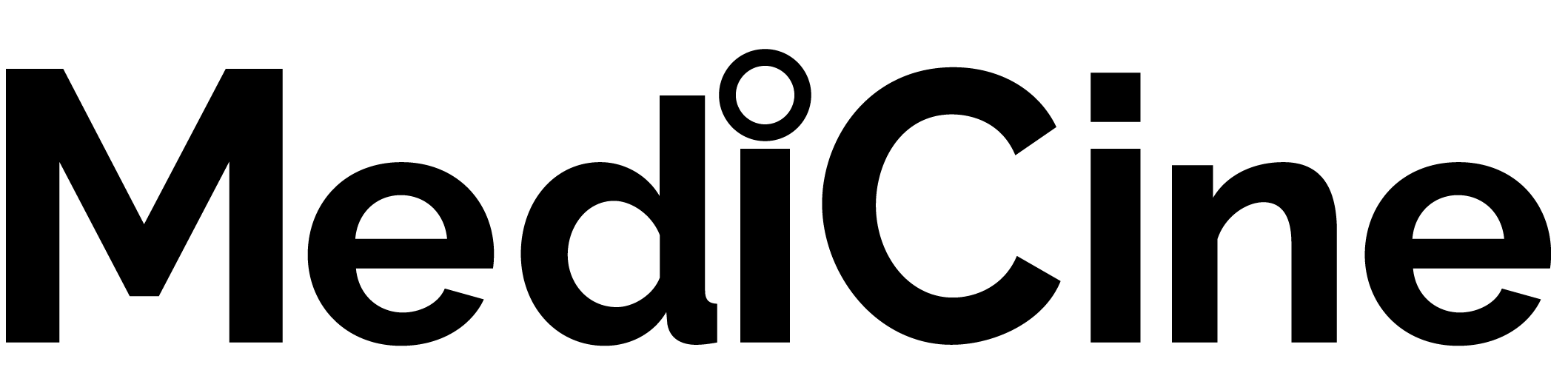
Issue 51
Aug 2024
INSIGHTS
By Julius Fredens, Assistant Professor, Synthetic Biology Translational Research Programme, Department of Biochemistry, NUS Yong Loo Lin School of Medicine
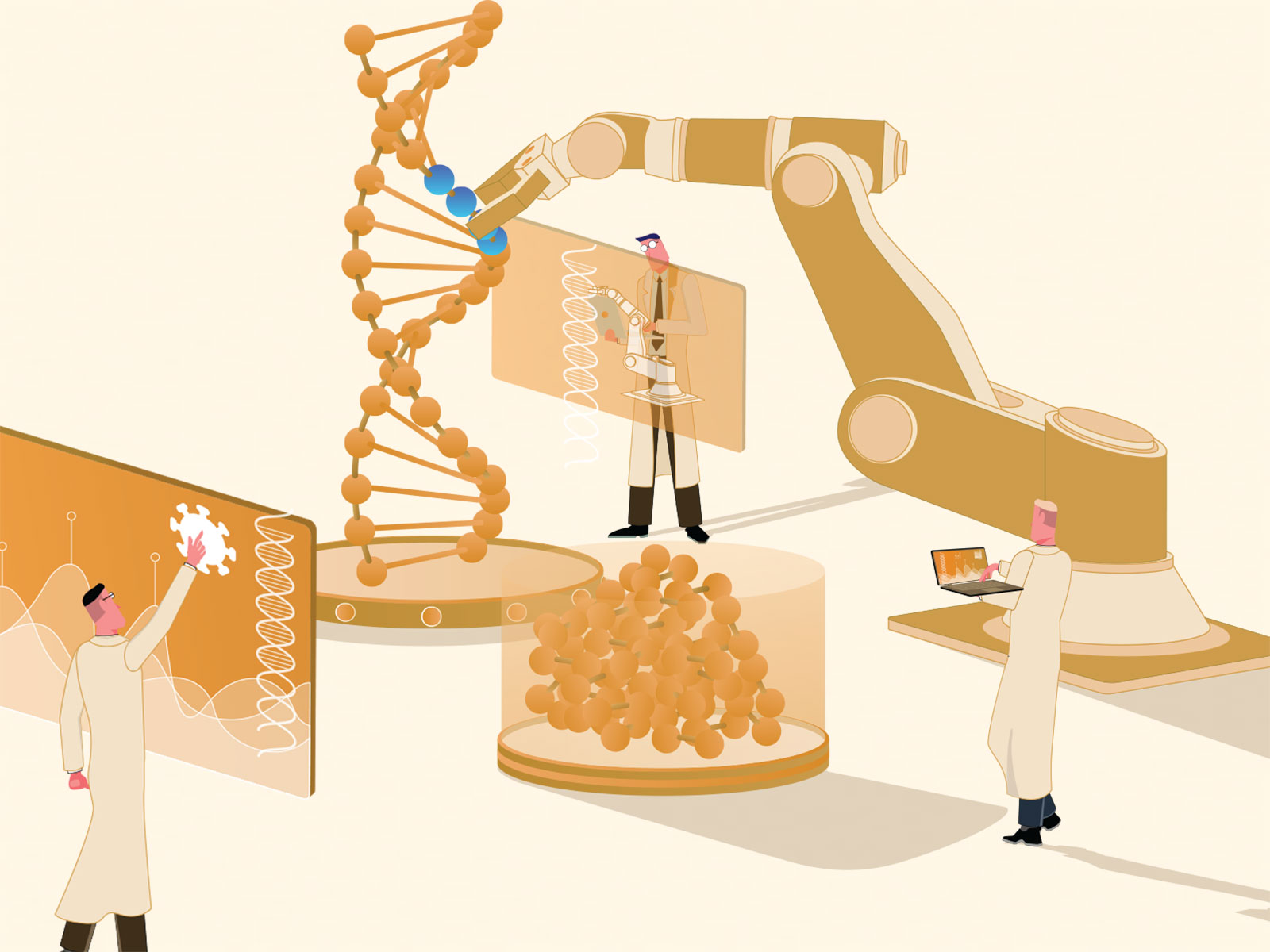
LAB LIFE: Right now is, perhaps, the most exciting time in history.
Life is crazy! Not just our experience of life; everyday routines, taking the MRT to work, seeing a rare bird, travelling to new countries, raising kids, and so on. No, the concept of something being alive is crazy: A tiny living cell is crazy complex, delicate, and somewhat unlikely. Yet, we are so used to being surrounded by excessive varieties of living organisms that we easily forget how intriguing life is. And we may only notice life is absent, for example when seeing photos of a desert, an alien planet, or a cleanroom in a laboratory.
Our view on life has experienced a tectonic shift from holistic to molecular over the past few centuries. For millennia, only useful organisms got any serious attention, but in the 1700s, naturalists began to study the broad variety of lifeforms surrounding them. Swedish botanist, Linnaeus, systematically mapped all plants and animals available to him, thereby implying a connection between all life forms. A century later, Darwin, another naturalist, dared looking further and suggesting that the spectacular diversity of life could have evolved from a single ancestor. Concurrently, an Austrian monk, Mendel, was inferring from his pea plant breeding that the chance of genetic traits is something calculable. Biology was becoming more exact.
While the above studies and theories started changing the way we humans look at life around us, the work was fairly abstract and the underlying mechanisms remained elusive. But all this changed dramatically overnight in 1953 and biology instantly became a highly molecular discipline. The smoking gun was the DNA double helix, described by Watson and Crick, partly by grabbing data from other researchers (without permission). Fantastically, this structure immediately enabled them to put forward a unifying theory of how cells can store and distribute genetic information when they divide.
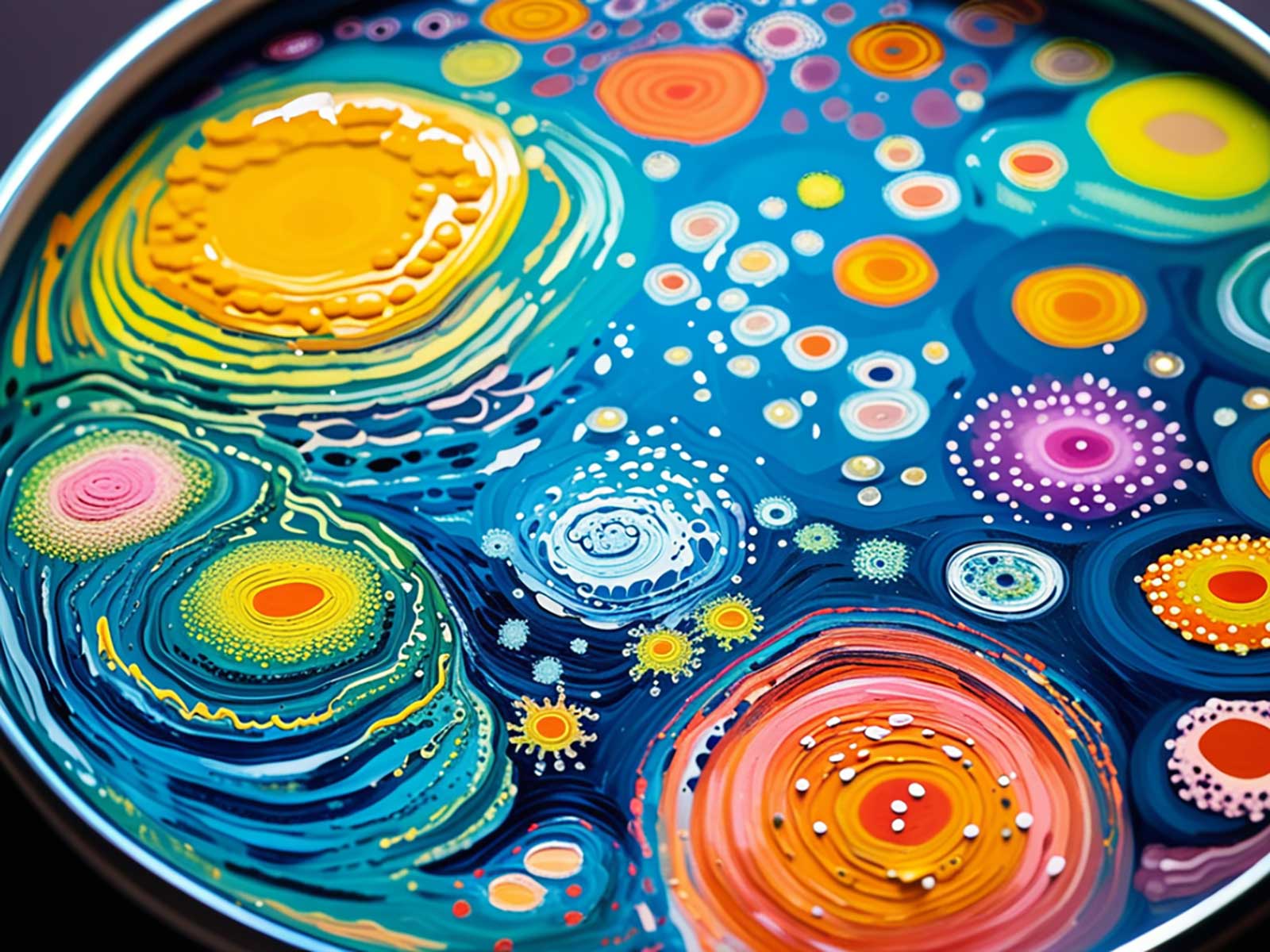
Cells growing in a petri dish in the lab. AI-enhanced with Imagine.art.
Despite the extraordinary complexity of life, the fundamental premise is simple: One becomes two. Here, biology differs from the other natural sciences in that the specimen can self-replicate and consistently multiply. I was many years into my training as a molecular biologist before I really appreciated this difference: Chemists must consider the quantity of product needed from a synthesis reaction. For biologists, on the other hand, quantity is merely a function of time—provided the all-important ability to recreate the right growth conditions. A living cell may be fragile, but its ability to multiply and adapt makes life tremendously powerful.
As soon as the structure of the DNA double helix was solved, the work to decipher the genetic code began. Quickly, this revealed that the 20 canonical amino acids are encoded by 64 codons and there therefore is quite a bit of redundancy in the genetic code, rather like computer coders like it in software. The most astonishing realisation, however, was that the same genetic code is conserved across almost all species in our biosphere despite almost 4 billion years of evolution.
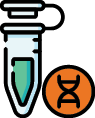
Fascinating as it is, the explanation is simple: the decoding of genetic information is the most vital function of the cell—and the sacred bond between genetic information and phenotype is the key to evolution. Once the code was established in the primordial cell, it became a fundamental piece of evolving life and, ironically, remained the only element unchanged over the course of evolution.
Working in the exciting field of synthetic biology research, I was fortunate enough to get the chance to create a synthetic genome and rewrite the genetic code, like a computer code or translating a book. With a group of enthusiastic colleagues, I developed several alternative genetic codes that explored the redundancy to faithfully encode the 20 amino acids from less than 64 codons. A few small tests in our favourite bacterium, Escherichia coli or better known as E. coli, were encouraging and we started thinking ‘some of these new genetic codes—the first in 4 billion years—might actually work’.
So, we redesigned the 4 million base pair E. coli genome and ordered it from a factory in 400 pieces of DNA, produced by chemical synthesis. It took us a few years to puzzle all the pieces together and stepwise replace the whole genome in the living E. coli. With only a handful of corrections to our original design, we ended up with an organism that looked and behaved like the good old E. coli but was running on a synthetic genome with a new genetic code. The transition from dead to alive is truly fascinating: From 1-2 mg of chemically synthetised DNA—a finite amount produced by an entirely non-biological means—into a genome, controlling a living organism that can replicate exponentially for years to come and easily outlive all of us, who designed and constructed it.
With the ability to design and build synthetic genomes, life has become functionally programmable. While this is being exploited for useful applications, the curiosity-driven research field starts eyeing opportunities for building synthetic organisms. Right now is, perhaps, the most exciting time in history for creating life in the lab.
More from this issue
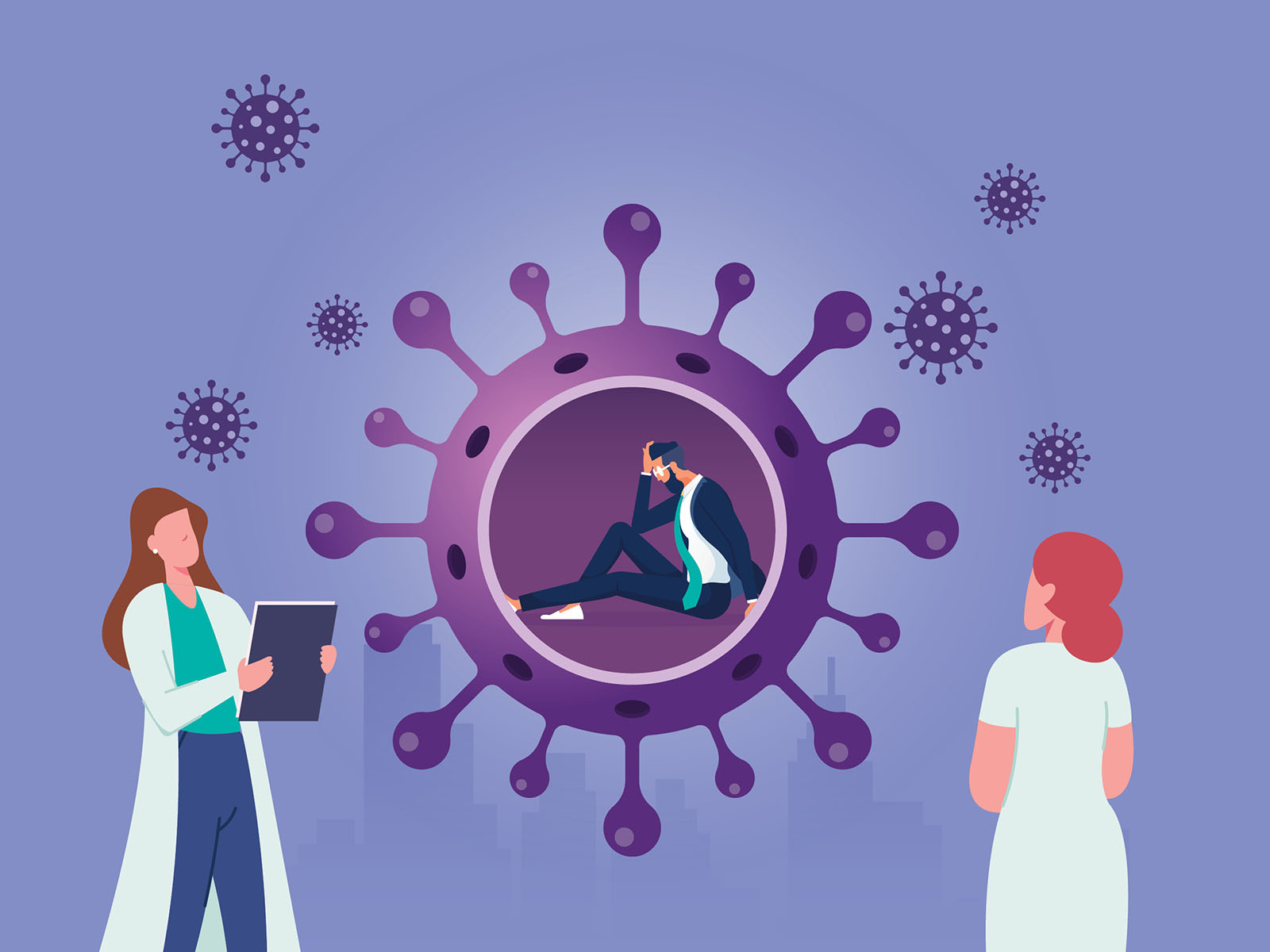
ETHICALLY SPEAKING
What Prompts People to Deliberately Expose Themselves to an Infection?
AFFAIRS OF THE HEART
Advancing Wellness: The Power of Human-Digital Collaboration in Chronic Illness Care
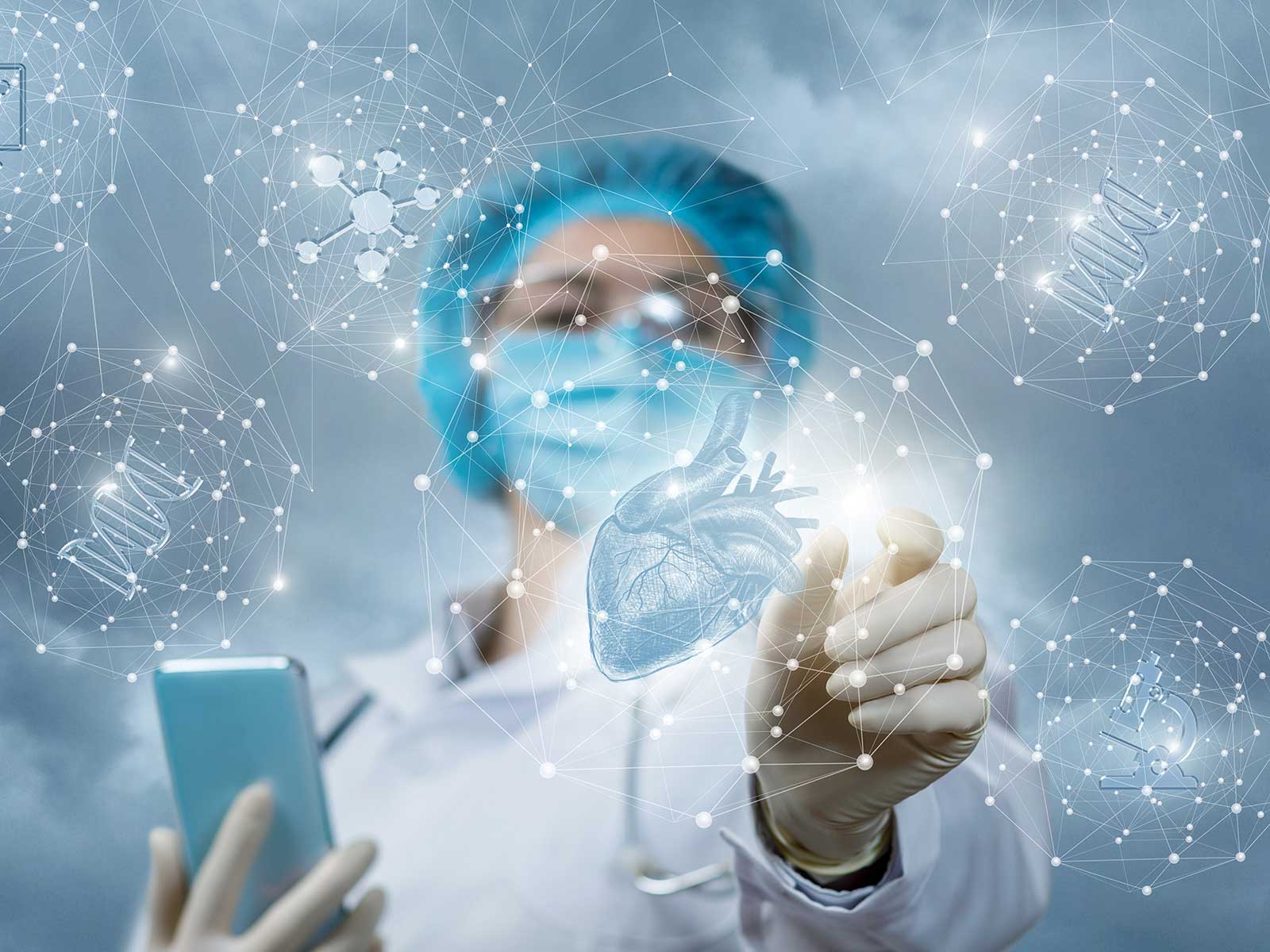
PEOPLE OF NUS MEDICINE
Leading Research Like Conducting an Orchestra
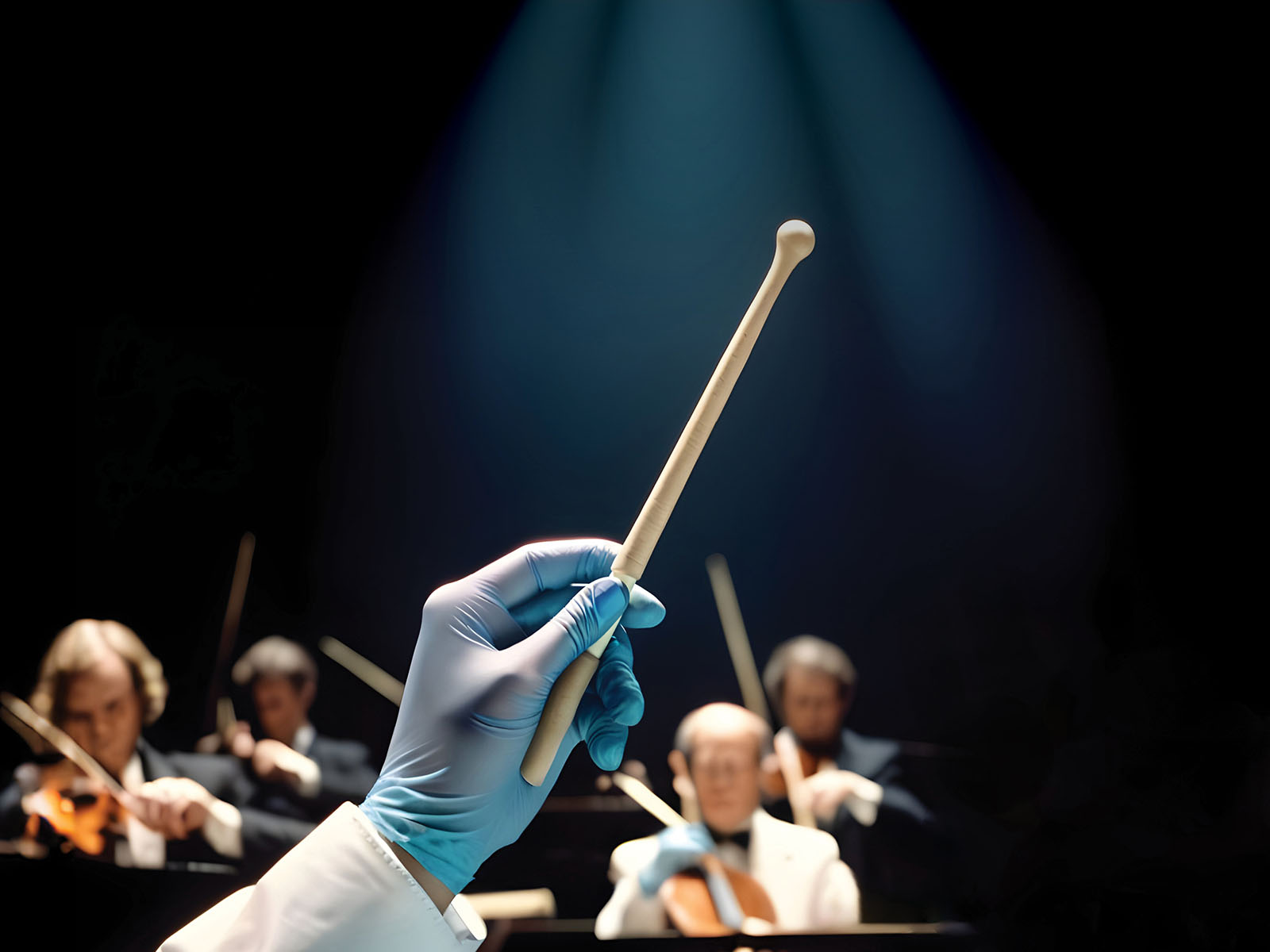