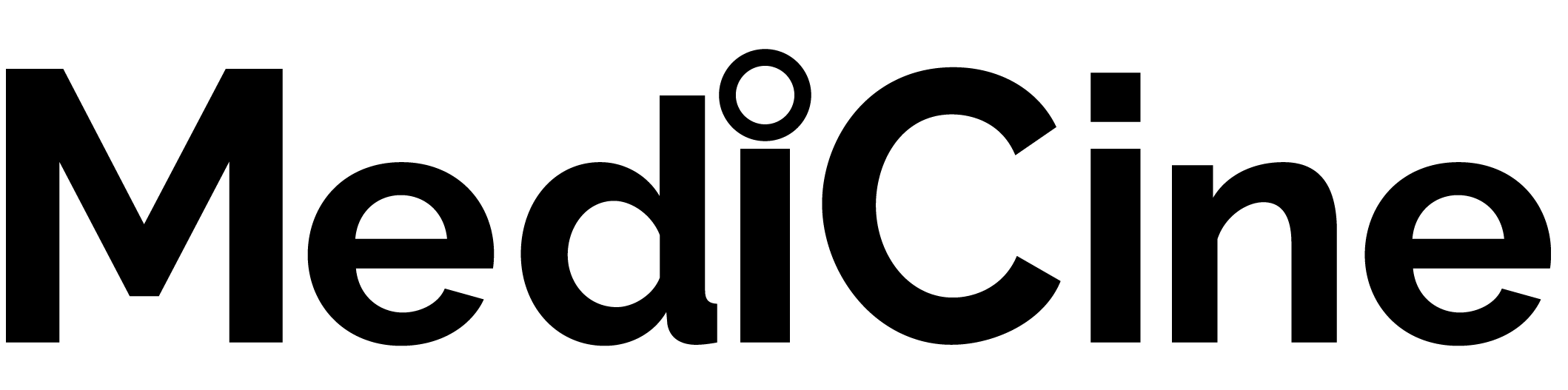
Issue 51
Aug 2024
A WORLD IN A GRAIN OF SAND
By Dr Jaishree Tripathi, Lecturer, Department of Microbiology & Immunology, NUS Yong Loo Lin School of Medicine
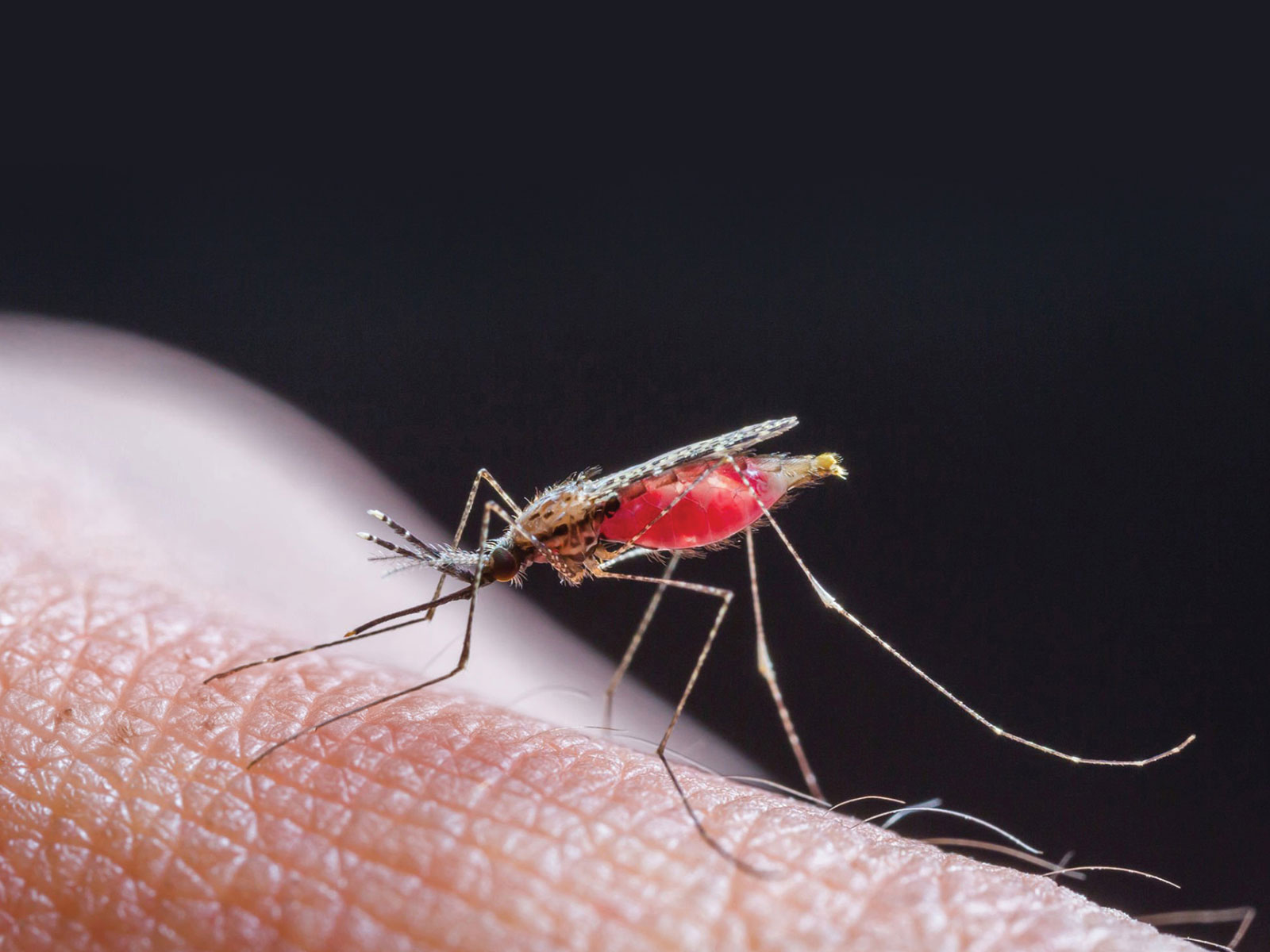
The fight against malaria is emblematic of the broader struggle against infectious diseases—a reminder that even the smallest pathogens can have outsized impacts on human health. In the poetic words of William Blake, "To see a world in a grain of sand," we are encouraged to find the extraordinary within the ordinary. This perspective is particularly relevant in the context of malaria, a disease caused by microscopic, single-celled parasites that has had a profound and enduring impact on humanity. Despite significant progress, the battle against malaria is far from over, with new challenges emerging and demanding innovative solutions.
The scale of the problem
Malaria remains one of the world’s most pressing public health issues, with an estimated 249 million cases and 608,000 deaths reported globally in 20221. Unfortunately, the majority of these fatalities occur in sub-Saharan Africa, with children under the age of 5 being affected primarily. Malaria is caused by unicellular protozoan parasites belonging to the genus Plasmodium which are transmitted through the bites of infected, female Anopheles mosquitoes. Historically, 4 species of Plasmodium were known to infect humans— P. falciparum, P. vivax, P. ovale, and P. malariae. Worryingly, this list has grown in recent years, as new zoonotic malaria infections caused by simian malaria parasites, P. knowlesi and P. cynomolgi, are discovered in humans in Southeast Asia2–4.
Latest progress
Significant strides have been made in the fight against malaria over the past 2 decades. The global incidence of malaria has declined from 81 per 1,000 population at risk in the year 2000 to 58 per 1,000 population at risk in 20221. This is largely due to concerted efforts in vector control (through bed nets treated with insecticides and indoor spraying), improved diagnostic techniques (such as rapid diagnostic tests or RDTs), and the widespread use of artemisinin-based combination therapies (ACTs). More recently, 2 malaria vaccines, RTS,S/AS01 (Mosquirix) and R21/Matrix-M, were recommended by the World Health Organization and are planned for rollout in several malaria endemic regions. Each of these malaria prevention and treatment arms plays a crucial role in reducing malaria transmission rates and improving outcomes for those infected. Nonetheless, multiple challenges still remain to be tackled to achieve malaria eradication.
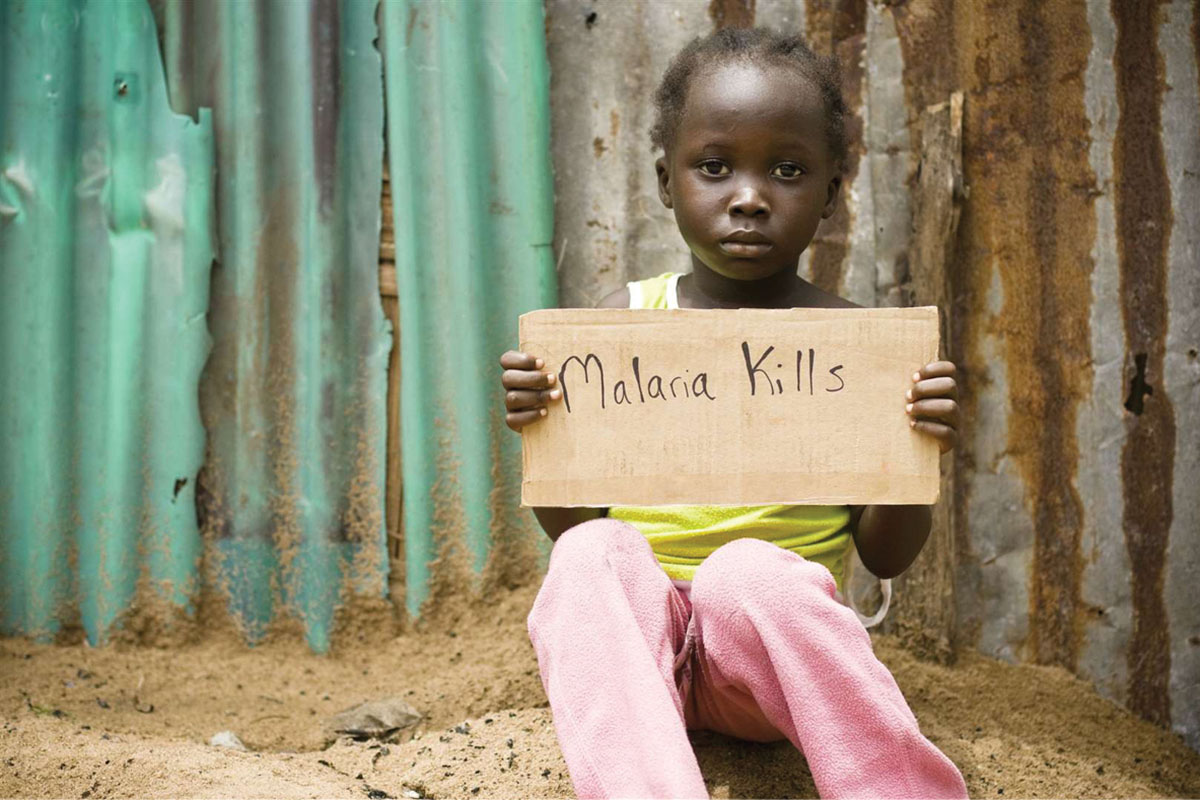
Malaria is one of the leading causes of child mortality in Africa.
Current challenges
The diversity of malaria parasites is a key factor in their resilience and survival. Different species of Plasmodium each has unique characteristics that complicate eradication efforts. For instance, P. falciparum‘s rapid development of drug resistance5,6, P. vivax‘s ability to form dormant liver stages (hypnozoites)7,8, and the varying geographical distributions and transmission patterns of each species create a multifaceted challenge. This genetic and biological diversity enables the parasites to adapt to various environmental pressures, evade host immune responses, and withstand control measures.
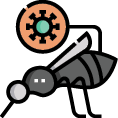
249 million
Malaria cases and
608,000
deaths are reported globally in 2022
Global incidence of malaria declined from 81 to
58
PER 1,000
population at risk from 2000 to 2022
• |
A specific example of this adaptability is antigenic variation, particularly in P. falciparum9,10. The parasite can alter the proteins expressed on the surface of infected red blood cells (RBC), notably through the var gene family, which encodes for several PfEMP1 proteins. This antigenic variation allows the parasite to evade the host’s immune system by continually changing its surface antigens, making it difficult for the immune system to recognise and attack the parasite effectively. This ability to switch between different antigenic forms is a significant obstacle in developing effective vaccines and treatments, as it helps the parasite persist in the host and spread to new individuals. |
• |
Widespread natural genetic polymorphisms also contribute to the parasite’s adaptability11,12. For instance, variations in the pfcrt gene are associated with chloroquine resistance, while mutations in the dhfr and dhps genes confer resistance to sulfadoxine-pyrimethamine. These genetic changes allow the parasites to survive despite the presence of drugs designed to eliminate them. P. falciparum has developed resistance to nearly every antimalarial drug used over the past century, from chloroquine and sulfadoxine-pyrimethamine to, more recently, artemisinin. The latter is of significant concern because ACTs are the cornerstone of current malaria treatment protocols. Artemisinin resistance, first identified in Southeast Asia, is characterised by a delayed clearance of parasites from the bloodstream and is now widely spread in countries such as Cambodia, Laos, Thailand, Myanmar and Bangladesh13. This resistance is often mediated by mutations in the kelch13 gene12, which reduce the efficacy of artemisinin. The spread of these resistant strains to Africa, where the malaria burden is highest, poses a dire threat to global malaria control efforts14. Researchers are now focusing on understanding molecular mechanisms underlying resistance to artemisinin15,16, and developing new drugs and combination therapies that can overcome this resistance, but this is a complex and ongoing challenge. Furthermore, transcriptional variation17–19, such as changes in gene expression levels in response to environmental conditions, enhances the parasite’s ability to thrive in diverse environments. For example, the transcriptional upregulation of heat shock proteins can help the parasite withstand the febrile conditions in a human host20. More recently, our research has shown that several of the parasite’s genes crucial for RBC invasion exhibit significant transcriptional variation17 and may explain the generally low efficacy of most invasion-blocking vaccines designed in the past. |
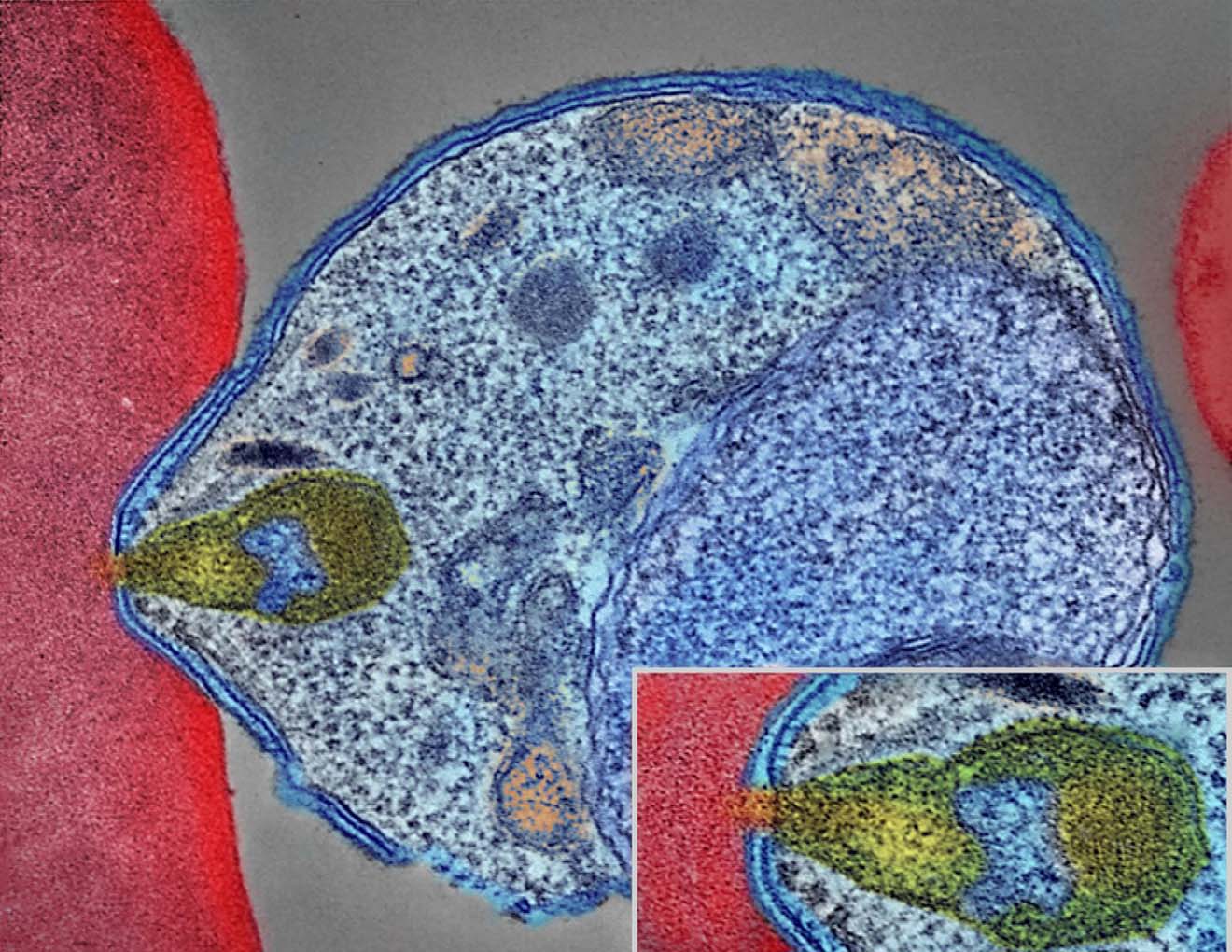
Colourised electron micrograph showing malaria parasite (right, blue) attaching to a human red blood cell. The inset shows a detail of the attachment point at higher magnification. Credit: NIAID.
• |
Another major hurdle in malaria eradication is the presence of dormant liver stages, or hypnozoites, in P. vivax21 and P. ovale infections. Outside of Africa, P. vivax is the dominant species with widespread prevalence in South America, Asia and Western Pacific regions. These hypnozoites can remain latent in the liver for months or even years before reactivating and causing relapses. This characteristic makes P. vivax malaria particularly difficult to eliminate because standard blood-stage treatments do not affect these dormant forms. The only approved drug for targeting hypnozoites is primaquine, but its use is limited due to the risk of severe hemolysis in individuals with glucose-6-phosphate dehydrogenase (G6PD) deficiency, a common genetic condition in malaria-endemic regions22. To address this, researchers are investigating safer and more effective alternatives. Tafenoquine, a new drug approved by the Food and Drug Administration (FDA) in 2018 for the radical cure of P. vivax malaria, offers a single-dose cure for the liver stage of P. vivax malaria and has shown promise in clinical trials. However, like primaquine, tafenoquine also poses a risk for individuals with G6PD deficiency, necessitating screening before use. |
• |
Vector Control and Surveillance. Beyond drug resistance and hypnozoites, vector control remains a critical component of malaria prevention. The widespread use of insecticide-treated bed nets and indoor residual spraying has been instrumental in reducing malaria transmission. However, mosquitoes are also evolving, with resistance to commonly used insecticides such as pyrethroids becoming increasingly prevalent. This necessitates the development of new insecticides and alternative strategies, such as biological control agents and genetic modifications of mosquito populations. Moreover, robust surveillance systems are essential for monitoring malaria transmission and the emergence of drug-resistant strains. Enhanced diagnostic techniques, including molecular tools for detecting low-level parasitemia and resistance markers, are crucial for timely and effective intervention. The use of geographic information systems (GIS) and mobile technology has improved the ability to track and respond to outbreaks in real-time, enabling more targeted and efficient control measures. |
The way forward
While the challenges are formidable, the future of malaria control and eventual eradication holds promise. Collaborative efforts between governments, international organisations, researchers, and communities are vital to sustaining progress. Continued investment in research and development is essential for discovering new drugs, vaccines, and vector control methods. Additionally, addressing the social determinants of health, such as poverty, education, and access to healthcare, is crucial for reducing the malaria burden.
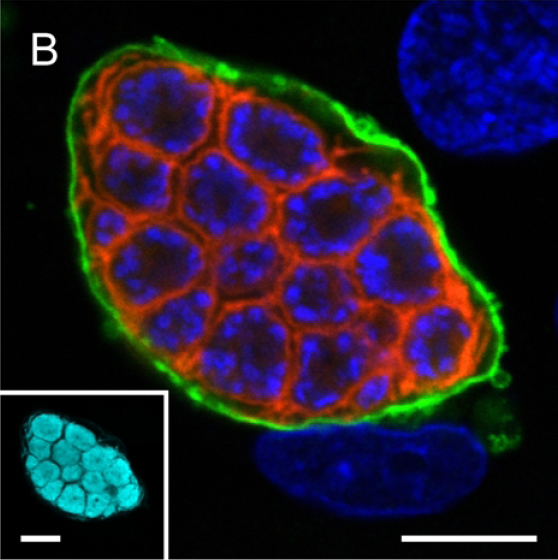
A confocal microscopy image of liver stage of malaria parasite (P. berghei) depicting parasite plasma membrane in red, nuclei in blue and parasitophorous vacuole membrane in green. The inset shows parasite cytoplasm labelled using a transgenically expressed protein (cyan) (Ref: Graewe et al, Plos Pathogens, 2011).
The story of malaria is a testament to the complexities and triumphs of global health. Like a world seen in a grain of sand, the intricacies of malaria—from the molecular mechanisms of drug resistance to the behavioural ecology of mosquito vectors—reveal a microcosm of the broader challenges faced in combating infectious diseases. The lessons learned from this ongoing battle will undoubtedly inform future efforts to address other emerging and re-emerging pathogens.
In conclusion, the fight against malaria encapsulates the essence of Blake’s poetic vision: finding significance and meaning in the minute details. As we continue to unravel the mysteries of this ancient scourge, each discovery, no matter how small, brings us closer to a world free of malaria. The path ahead requires perseverance, innovation, and a steadfast commitment to overcoming the challenges that lie in our way. Through collective action and scientific advancement, a world without malaria is within our grasp—a testament to the extraordinary potential found within the grains of sand.
The story of malaria is a testament to the complexities and triumphs of global health. As we continue to unravel the mysteries of this ancient scourge, each discovery, no matter how small, brings us closer to a world free of malaria. The path ahead requires perseverance, innovation, and a steadfast commitment to overcoming the challenges that lie in our way.”
WHO. World Malaria Report 2023. (2023).
Dian, N. D., Rahim, M. A. F. A., Chan, S. & Idris, Z. M. Non-Human Primate Malaria Infections: A Review on the Epidemiology in Malaysia. International Journal of Environmental Research and Public Health vol. 19 at https://doi.org/10.3390/ijerph19137888 (2022).
Imwong, M. et al. Asymptomatic natural human infections with the simian malaria parasites plasmodium cynomolgi and plasmodium knowlesi. J. Infect. Dis. 219, 695–702 (2019).
Yap, N. J. et al. Natural Human Infections with Plasmodium cynomolgi, P. inui, and 4 other Simian Malaria Parasites, Malaysia. Emerg. Infect. Dis. 27, 2187–2191 (2021).
Fairhurst, R. M. & Dondorp, A. M. Artemisinin-Resistant Plasmodium falciparum Malaria. Emerg. Infect. 10 4, 409–429 (2016).
Thu, A. M., Phyo, A. P., Landier, J., Parker, D. M. & Nosten, F. H. Combating multidrug-resistant Plasmodium falciparum malaria. FEBS J. 284, 2569–2578 (2017).
Taylor, A. R. et al. Resolving the cause of recurrent Plasmodium vivax malaria probabilistically. Nat. Commun. 10, 1–11 (2019).
Hulden, L. & Hulden, L. Activation of the hypnozoite : a part of Plasmodium vivax life cycle and survival. Malar. J. 10, 1–6 (2011).
Duraisingh, M. T. & Horn, D. Epigenetic Regulation of Virulence Gene Expression in Parasitic Protozoa. Cell Host Microbe 19, 629–640 (2016).
Guizetti, J. & Scherf, A. Silence, activate, poise and switch! Mechanisms of antigenic variation in Plasmodium falciparum. Cell. Microbiol. 15, 718–726 (2013).
Neafsey, D. E. et al. Genome-wide SNP genotyping highlights the role of natural selection in Plasmodium falciparum population divergence. Genome Biol. 9, R171 (2008).
Miotto, O. et al. Genetic architecture of artemisinin-resistant Plasmodium falciparum. Nat. Genet. 47, (2015).
van der Pluijm, R. W. et al. Determinants of dihydroartemisinin-piperaquine treatment failure in Plasmodium falciparum malaria in Cambodia, Thailand, and Vietnam: a prospective clinical, pharmacological, and genetic study. Lancet Infect. Dis. 19, 952–961 (2019).
Conrad, M. D. & Rosenthal, P. J. Antimalarial drug resistance in Africa: the calm before the storm? Lancet Infect. Dis. 19, e338–e351 (2019).
Zhu, L. et al. The origins of malaria artemisinin resistance defined by a genetic and transcriptomic background. Nat. Commun. 9, 1–13 (2018).
Rocamora, F. et al. Oxidative stress and protein damage responses mediate artemisinin resistance in malaria parasites. PLoS Pathog. 14, (2018).
Tripathi, J., Zhu, L., Nayak, S., Stoklasa, M. & Bozdech, Z. Stochastic expression of invasion genes in Plasmodium falciparum schizonts. Nat. Commun. 13, 1–15 (2022).
Walzer, K. A., Fradin, H., Emerson, L. Y., Corcoran, D. L. & Chi, J. T. Latent transcriptional variations of individual Plasmodium falciparum uncovered by single-cell RNA-seq and fluorescence imaging. PLoS Genet. 15, 1–26 (2019).
Tarr, S. J. et al. Schizont transcriptome variation among clinical isolates and laboratory-adapted clones of the malaria parasite Plasmodium falciparum. BMC Genomics 19, 1–13 (2018).
Tintó-Font, E. et al. A heat-shock response regulated by the PfAP2-HS transcription factor protects human malaria parasites from febrile temperatures. Nature Microbiology vol. 6 (2021).
Wells, T. N. C., Burrows, J. N. & Baird, J. K. Targeting the hypnozoite reservoir of Plasmodium vivax: the hidden obstacle to malaria elimination. Trends Parasitol. 26, 145–51 (2010).
Kwiatkowski, D. P. How malaria has affected the human genome and what human genetics can teach us about malaria. Am. J. Hum. Genet. 77, 171–92 (2005).
More from this issue
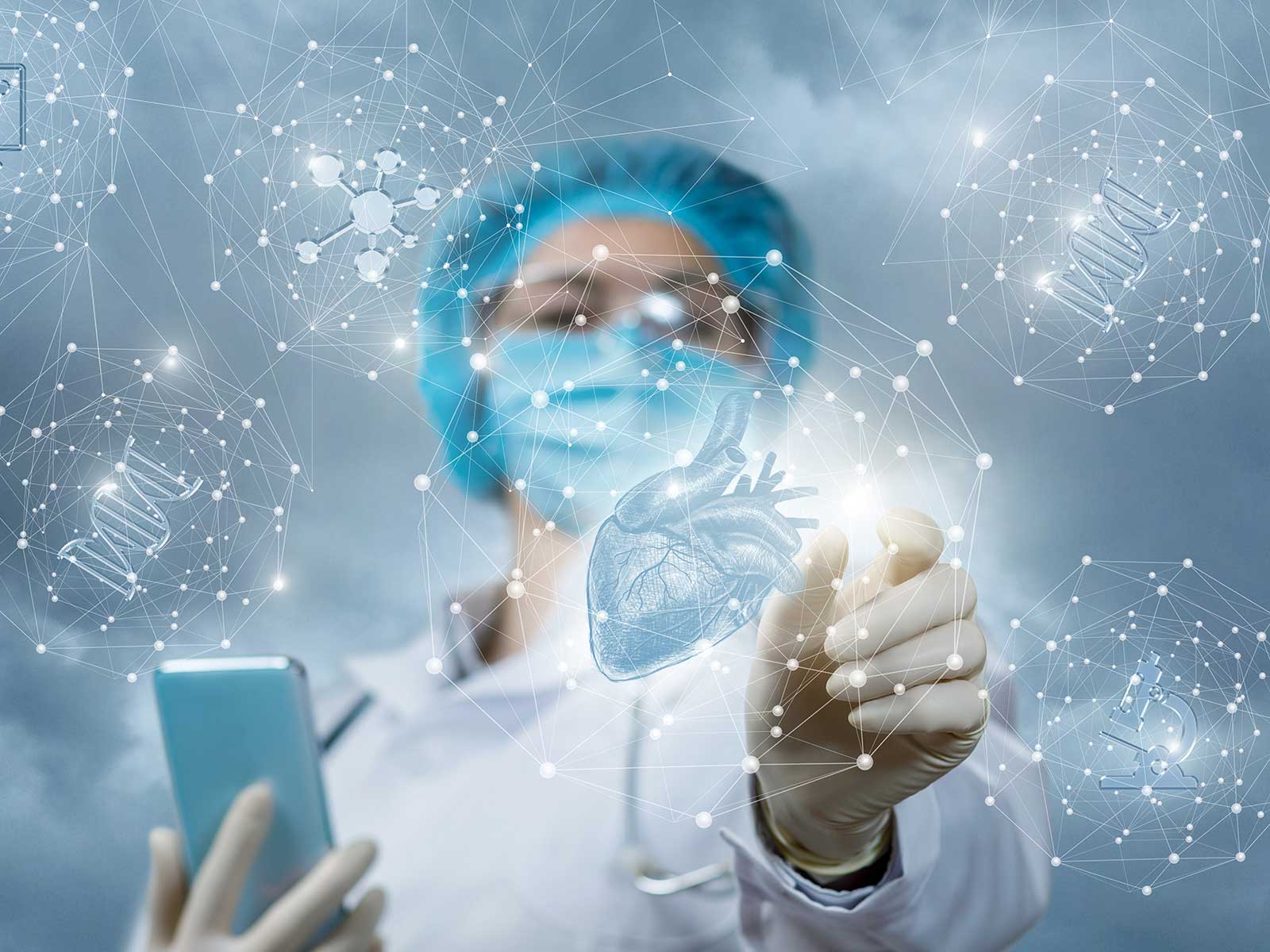
AFFAIRS OF THE HEART
Advancing Wellness: The Power of Human-Digital Collaboration in Chronic Illness Care
STUDENT MATTERS
Public Health Service
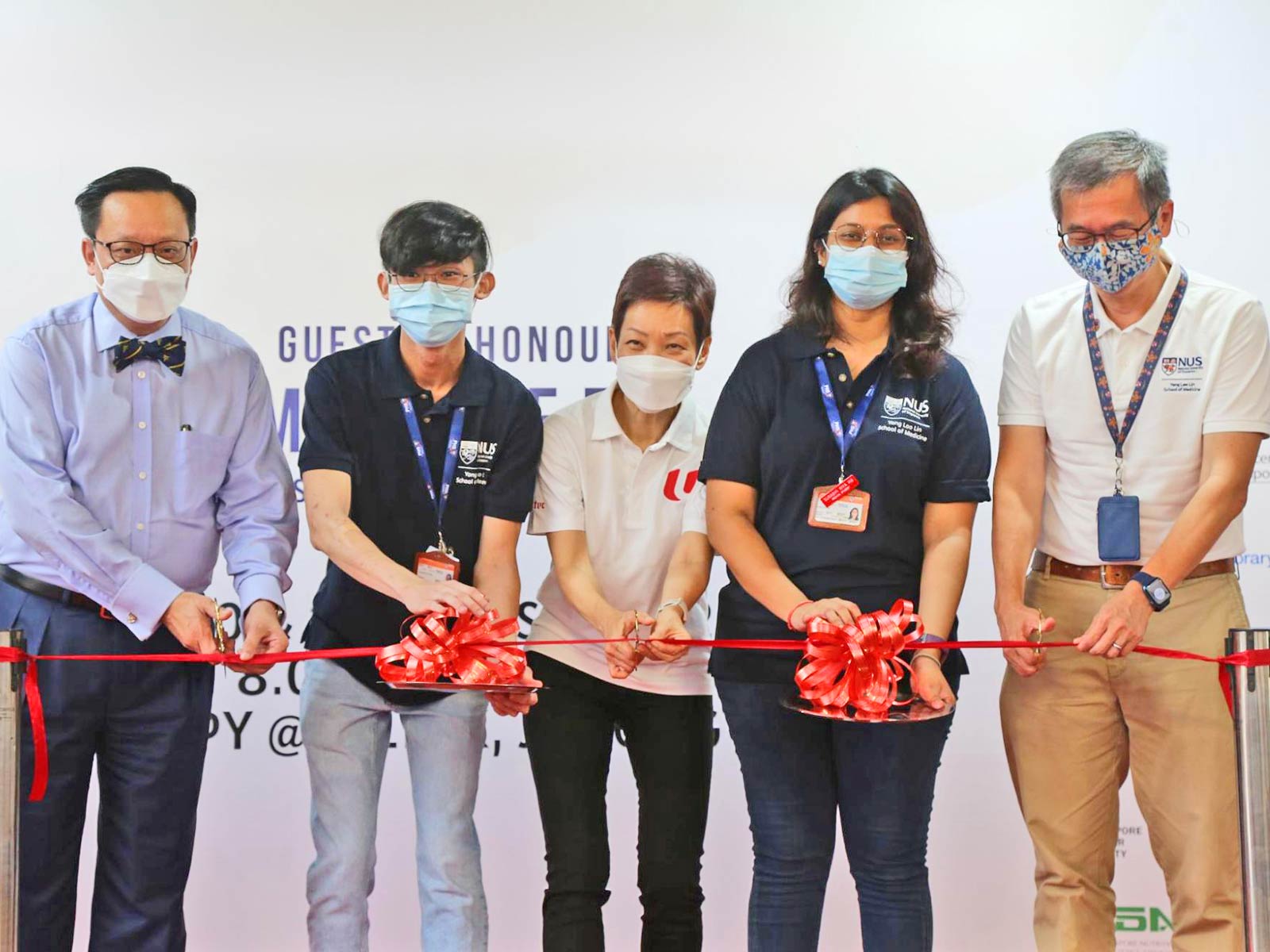
THE LAST MILE
When Your Patient Dies by Suicide
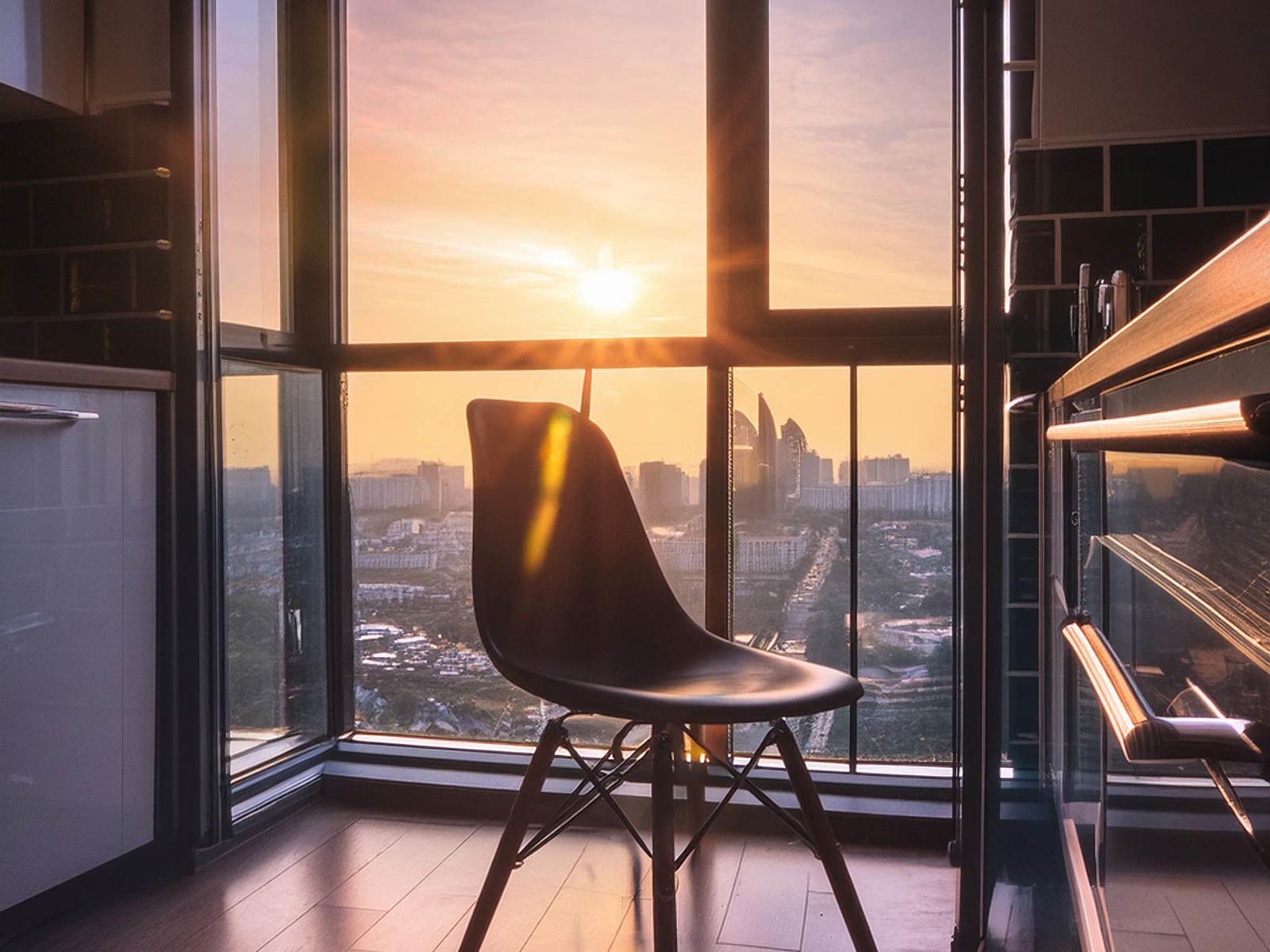