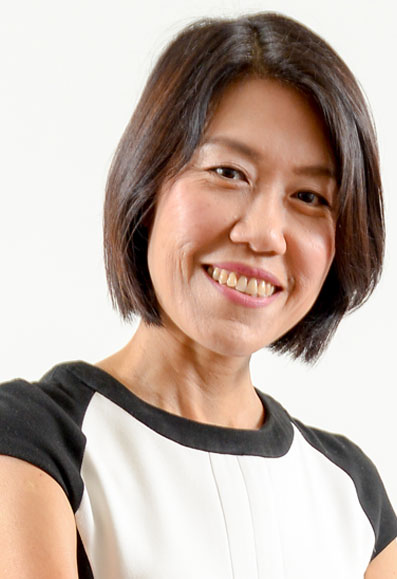
Affiliations
Associate Professor, Department of Biochemistry, Yong Loo Lin School of Medicine, NUS.
Co-Director, Infectious Diseases Translational Research Program, Yong Loo Lin School of Medicine, NUS.
Assistant Dean, Equal Opportunities and Career Development, Yong Loo Lin School of Medicine, NUS.
Biodata
Yunn-Hwen Gan graduated from Purdue University with a B.Sc. (Honours) in Molecular Biology and University of Wisconsin-Madison with a Ph.D. in medical microbiology and immunology. She is a world leading researcher in melioidosis, a disease primarily in the tropics caused by the bacterium Burkholderia pseudomallei. She established the first mucosal animal model in 2002 and had helped define the field by examining pathogen’s virulence as well as the host immune response to the disease. Her lab discovered a regulatory cascade that coordinately controlled two bacterial secretion systems which are absolutely critical for bacterial virulence. Other seminal findings include the discovery that glutathione deficiency is the reason for increased disease risk to melioidosis for Type 2 diabetic patients.
Her current research focuses on Klebsiella induced liver abscess, a prominent disease in Asia, particularly in regions of China, Taiwan, Hong Kong, Singapore and South Korea. Her work involves identifying bacterial virulence factors of hypervirulent Klebsiella pneumoniae responsible for causing liver abscess. Her team examines host and bacterial factors that affect gut colonization and translocation. Her recent works have identified the alarming trend of the convergence of multidrug resistance and hypervirulence in K. pneumoniae in Singapore’s hospital settings. Her ongoing research investigates how antibiotic resistance genes on highly evolved and adapted plasmids dominant in clinical bacterial isolates are spreading among bacterial populations, and strategies to stop the spread.
She has also established multidisciplinary collaborations with chemists, clinicians and computational biologists to examine novel strategies to treat antibiotic resistant Enterobacteriaceae bacteria. One of such strategies is to establish a synthetic commensal community of bacteria to be used as probiotics for decolonization from the gut. Another strategy is to partner with AI scientists to employ synergy testing of FDA-approved compounds, including those with no known antibacterial activity on their own, on multidrug resistant bacteria.
Research Interest
- Novel strategies for targeting multidrug resistant enteric bacteria.
- Multidrug resistance plasmid transmission in clinical Gram-negative bacteria.
- Bacterial sensing of host environment by two component sensor/regulator systems.
- Identifying risk factors for Klebsiella induced liver abscess.
- The influence of microbiome on Klebsiella pneumoniae gut colonization
Projects
Klebsiella Induced Liver Abscess (KLA)
KLA is the single most important cause of monomicrobial liver abscess and its subsequent complications in many parts of Asia, including Singapore. We examine bacterial factors and the ensuing host immune responses in the encounter between hypervirulent and its mammalian host. It is believed that many infections initiate from bacteria colonized in the intestines as they translocate across the intestinal barrier and travel via the hepatic portal vein to the liver. We are interested in examining both bacterial and host factors at play at each stage of the infection, including during the colonization stage. We routinely use a hypervirulent strain isolated from a liver abscess patient designated as a reference strain, SGH10, for our investigations. Using murine colonization and infection models, as well as primary human intestinal organoids, we hope to understand when and how the bacteria transition from a gut colonizer to a potentially deadly pathogen.
Figure 1. Scanning Electron Microscope (SEM) images of SGH10 WT strain at 40,000X magnification (left) and SGH10ΔwcaJ capsule mutant strain at 60,000X magnification (right). (Publication link : 🔗)
Figure 2. Transcriptional phenotypic switch between T3F and capsule hypermucoviscosity in response to changing iron levels. (A) Low iron condition results in hypermucoid capsule production and repressed T3F, leading to low biofilm formation and cell adhesion. (B) Iron-rich condition results in expression of T3F and downregulation of capule mucoviscosity, leading to high biofilm formation and cell adhesion. (Publication link : 🔗)
Figure 3. Genomic Islands GIE492 and ICEKp10 enable hypervirulent K. pneumoniae to kill several commensal bacterial taxa during interspecies interactions in the gut. Thus, acquisition of GIE492 and ICEKp10 could enable better carriage in host populations and explain the dominance of the CG23-I HvKp lineage. (Publication link : 🔗)
Multidrug resistance plasmid transmission in Klebsiella pneumoniae
We are interested in understanding the factors driving the transmission of carbapenem-resistance and multi-drug resistance plasmids in hospitals. We track plasmid and resistance genes dominance over time in a multi-centre collaboration, then apply molecular genetics to determine plasmid and bacterial host factors favouring spread and stability of carriage. In particular, we focus on the emergence of hypervirulent and carbapenem-resistant K. pneumoniae strains and aim to define factors and conditions leading to this convergence.
Figure 4. Conjugation frequency of pKPC2 and pNDM1 among various Enterobacterales donor-recipient pairs. (A) Conjugation frequency of pKPC2 and pNDM1 from K. pneumoniae SGH10 plasmid donor strain to a panel of Enterobacterales recipient strains; and B) from the panel of Enterobacterales plasmid donor strains to K. pneumoniae SGH10 recipient strain. Each symbol represents 1 experimental replicate with a total of 12 replicates. Data points that are not seen on the graphs indicate no detectable transconjugant. (Publication link : 🔗)
Novel strategies to target transmission of carbapenem-resistance in Enterobacterales
We engage in extensive collaborations with clinical colleagues, computational biologists, and chemists to develop strategies for combating carbapenem resistant Enterobacterales (CRE). Our efforts involve evaluating the efficacy and clinical potential of innovative synthetic antibacterial compounds and materials. Furthermore, we explore combinatorial therapy guided by AI platform to identify novel drug combinations capable of effectively eradicating CRE.
Figure 5. OIM1-6’s DNA binding properties and resulting DNA damage. (A) Flow cytometry analysis demonstrates picogreen displacement by OIM1-6 inside bacterial cytoplasm, indicating the DNA-binding properties of OIM1-6. Picogreen is a DNA binding dye with fluorescence level measured under Alexa Fluor 488 channel. (B) Confocal images showing the treatment of OIM1-6 leads to more foci formation in E. coli Gam-GFP strain as compared to the untreated cells. The hydrogen peroxide cells served as positive control with elevated level of foci formation. The presence of foci is indicative of double-stranded DNA breaks caused by the drug treatment. (Publication link : 🔗)
Figure 6. Workflow of the IDentif.AI-guided drug combinatorial study against CRE. Two clinical isolates from the most representative CRE species (hvKp ENT646 and E. coli C31) are investigated by IDentif.AI. The workflow begins by selecting 12 FDA-approved drugs, and they are individually assessed via dose-response experiment in vitro. Relevant concentration levels are selected. Subsequently, 155 OACD-designed combinations are experimentally validated, and the respective data are analyzed by IDentif.AI. Top combinations selected by the platform are comprehensively analyzed. (Publication link : 🔗)
Interaction of Burkholderia pseudomallei with host cells
B. pseudomallei is unique among bacterial pathogens in its ability to fuse host cells into multinucleated giant cells (MNGCs). Fusion is dependent on bacterial intracellular motility through the polymerization of actin into “actin comet tails” and the Type 6 Secretion System (T6SS5). We discovered that the process of cell fusion results in genomic instability and formation of micronuclei. Host cells sense danger brought on by unnatural cell fusion and this then triggers the cGAS-STING innate immune signalling pathway that ultimately leads to autophagic cell death. This could be the body’s defense against cellular transformation.
One of the routes of infection is through breaks in skin, such as those experienced by farmers with exposure to soil. We also examine the interaction of the bacteria with primary keratinocytes and the response of the host at this encounter.
Figure 7. MNGC formation in HepG2 liver epithelial cell. Bacteria (Burkholderia spp.) is shown in red, the cell periphery in green and the cell nuclei in blue. (Publication link : 🔗)
Movie 1. B. thailandensis infected multinucleated giant cell containing highly condensed mitotic-like chromosomes. Cell rounds up, initiates cytokinesis but eventually abort the process. HepG2 cells were infected with mApple fluorescent B. thailandensis (in Red) and stained with CellMask plasma membrane dye (in Magenta) and Hoechst 33342 DNA dye (in Blue).
Publications
- Goh KJ, Altuvia Y, Argaman L, Raz Y, Bar A, Lithgow T, Margalit H and Gan YH. (2024). RIL-seq reveals extensive involvement of small RNAs in virulence and capsule regulation in hypervirulent Klebsiella pneumoniae. Nucleic Acids Research. doi: 10.1093/nar/gkae440. 🔗
- Plum MTW, Cheung HC, Iscar PR, Chen Y, Gan YH and Basler M. (2024). Burkholderia thailandensis uses a type VI secretion system to lyse protrusions without triggering host cell responses. Cell Host and Microbe. doi: 10.1016/j.chom.2024.03.013. 🔗
- Tan YH, Arros P, Berrios-Pasten C, Wijaya I, Chu WHW, Chen Y, Cheam G, Naim ANM, Marcoleta AE, Ravikrishnan A, Nagarajan N, Lagos R and Gan YH. (2024). Hypervirulent Klebsiella pneumoniae employs genomic island encoded toxins against bacterial competitors in the gut. The ISME Journal. doi: 10.1093/ismejo/wrae054. 🔗
- Galvez-Silva M, Arros P, Berrios-Pasten C, Villamil A, Rodas PI, Araya I, Iglesias R, Araya P, Hormazabal JC, Bohle C, Chen Y, Gan YH, Chavez FP, Lagos R and Marcoleta AE (2024). Carbapenem-resistant hypervirulent ST23 Klebsiella pneumoniae with a highly transmissible dual-carbapenemase plasmid in Chile. Biological Research. doi: 10.1186/s40659-024-00485-2. 🔗
- Chang KC, Nagarajan N and Gan YH. (2024). Short-chain fatty acids of various lengths differentially inhibit Klebsiella pneumoniae and Enterobacteriaceae species. mSphere. doi: 10.1128/msphere.00781-23. 🔗
- Li M, You K, Wang P, Hooi L, Chen Y, Siah A, Tan SB, Teo J, Ng OT, Marimuthu K, Venkatachalam I, Blasiak A, Chow EKH, Ho D and Gan YH. (2024). Discovery of Broad-Spectrum Repurposed Drug Combinations Against Carbapenem-Resistant Enterobacteriaceae (CRE) Through Artificial Intelligence (AI)-driven Platform. Advanced Therapeutics. doi: 10.1002/adtp.202300332. 🔗
- Rojas D, Marcoleta AE, Galvez-Silva M, Varas MA, Diaz M, Hernandez M, Vargas C, Nourdin-Galindo G, Koch E, Saldivia P, Vielma J, Gan YH, Chen YH, Guiliani N and Chavez FP. (2024). Inorganic Polyphosphate Affects Biofilm Assembly, Capsule Formation, and Virulence of Hypervirulent ST23 Klebsiella pneumoniae. ACS Infectious Diseases. doi: 10.1021/acsinfecdis.3c00509. 🔗
- Chen Y, Yong M, Li M, Si Z, Koh CH, Lau P, Chang YW, Teo J, Chan-Park MB and Gan YH. (2023). A hydrophilic polyimidazolium antibiotic targeting the membrane of Gram-negative bacteria. Journal of Antimicrobial Chemotherapy. doi: 10.1093/jac/dkad274. 🔗
- Chu WHW, Tan YH, Tan SY, Chen Y, Yong M, Lye DC, Kalimuddin S, Archuleta S and Gan YH. (2023). Acquisition of regulator on virulence plasmid of hypervirulent Klebsiella allows bacterial lifestyle switch in response to iron. mBio. doi: 10.1128/mbio.01297-23. 🔗
- Yong M, Kok ZY, Koh CH, Zhong W, Ng JTY, Mu Y, Chan-Park MB and Gan YH. (2023). Membrane potential-dependent uptake of cationic oligoimidazolium mediates bacterial DNA damage and death. Antimicrobial Agents and Chemotherapy. doi: 10.1128/aac.00355-23. 🔗
- Kang JTL, Teo JJY, Bertrand D, Ng A, Ravikrishnan A, Yong M, Ng OT, Marimuthu K, Chen SL, Chng KR, Gan YH and Nagarajan N. (2022). Long-term ecological and evolutionary dynamics in the gut microbiomes of carbapenemase-producing Enterobacteriaceae colonized subjects. Nature Microbiology. doi: 10.1038/s41564-022-01221-w. 🔗
- Goncalves RA, Ku JWK, Zhang H, Salim T, Oo G, Zinn AA, Boothroyd C, Tang RMY, Gan CL, Gan YH, and Lam YM. (2022). Copper-Nanoparticle-Coated Fabrics for Rapid and Sustained Antibacterial Activity Applications. CS Appl. Nano Mater. doi: 10.1021/acsanm.2c02736. 🔗
- Yong M, Chen Y, Oo G, Chang KC, Chu WHW, Teo J, Venkatachalam I, Thevasagayam NM, Sridatta PSR, Koh V, Marcoleta AE, Chen H, Nagarajan N, Kalisvar M, Ng OT, and Gan YH. (2022). Dominant Carbapenemase-Encoding Plasmids in Clinical Enterobacterales Isolates and Hypervirulent Klebsiella pneumoniae, Singapore. Emerg Infect Dis 28, 1578-1588. 🔗
- Lim DRX, Chen Y, Ng LF, Gruber J, Gan YH. (2022). Glutathione catabolism by Enterobacteriaceae species to hydrogen sulfide adversely affects viability in host systems in the presence of 5’fluorodeoxyuridine. Mol Microbiol. doi: 10.1111/mmi.14893. 🔗
- Ku JWK and Gan YH. (2021). New roles for glutathione: Modulators of bacterial virulence and pathogenesis. Redox Biology 44, 102012. 🔗
- Molton JS, Lee R, Bertrand D, Ding Y, Kalimuddin S, Lye DC, Nagarajan N, Gan YH, Archuleta S. (2021). Stool metagenome analysis of patients with Klebisella pneumoniae liver abscess and their domestic partners. Int J Infect Dis 107, 1- 4. 🔗
- Zhong W, Shi Z, Mahadevegowda SH, Liu B, Zhang K, Koh CH, Ruan L, Chen Y, Zeden MS, Pee CJE, Marimuthu K, De PP, Ng OT, Zhu Y, Chi YR, Hammond PT, Yang L, Gan YH, Pethe K, Greenberg EP, Grundling A, and Chan-Park MB. (2020). Designer broad-spectrum polyimidazolium antibiotics. PNAS 117, 31376-31385. 🔗
- Hoong BYD, Gan YH, Liu H, and Chen ES. (2020). cGAS-STING pathway in oncogenesis and cancer therapeutics. Oncotarget 11, 2930-2955. 🔗
- Ku JWK, Chen Y, Lim BJW, Gasser S, Crasta KC, and Gan YH. (2020). Bacterial-induced cell fusion is a danger signal triggering cGAS–STING pathway via micronuclei formation. PNAS. 🔗
- Chng KR, Ghosh TS, Tan YH, Nandi T, Lee IR, Ng AHQ, Li C, Ravikrishnan A, Lim KM, Lye D, et al. (2020). Metagenome-wide association analysis identifies microbial determinants of post-antibiotic ecological recovery in the gut. Nat Ecol Evol. 🔗
- Qi G, Hu F, Kenry, Chong KC, Wu M, Gan YH, and Liu B. (2020). Bacterium‐Templated Polymer for Self‐Selective Ablation of Multidrug‐Resistant Bacteria. Advanced Functional Materials. 🔗
- Chen Y, Marimuthu K, Teo J, Venkatachalam I, Cherng BPZ, De Wang L, Prakki SRS, Xu W, Tan YH, Nguyen LC, Koh TH, Ng OT and Gan YH. (2020). Acquisition of Plasmid with Carbapenem-Resistance Gene blaKPC2 in Hypervirulent Klebsiella pneumoniae, Singapore. Emerg Infect Dis 26, 549-559. 🔗
- Si Z, Lim HW, Tay MYF, Du Y, Ruan L, Qiu H, Zamudio-Vazquez R, Reghu S, Chen Y, Tiong WS, Marimuthu PPDe, Ng OT, Zhu Y, Gan YH, Chi YR, Duan H, Bazan GC, Greenberg EP, Chan-Park MB, and Pethe K. (2020). A Glycosylated Cationic Block Poly(beta-peptide) Reverses Intrinsic Antibiotic Resistance in All ESKAPE Gram-Negative Bacteria. Angew Chem Int Ed Engl 59, 6819-6826. 🔗
- Tan YH, Chen Y, Chu WHW, Sham LT, and Gan YH. (2020). Cell envelope defects of different capsule-null mutants in K1 hypervirulent Klebsiella pneumoniae can affect bacterial pathogenesis. Mol Microbiol. 🔗
- Hoh CH, Tan YH, and Gan YH. (2019). Protective Role of Kupffer Cells and Macrophages in Klebsiella pneumoniae-Induced Liver Abscess Disease. Infect Immun 87. 🔗
- Ku JW, and Gan YH. (2019). Modulation of bacterial virulence and fitness by host glutathione. Curr Opin Microbiol 47, 8-13. 🔗
- M C Lam M, Wyres KL, Duchêne S, Wick RR, Judd LM, Gan YH, Hoh CH, Archuleta S, Molton JS, Kalimuddin S, Koh TH, Passet V, Brisse S, Holt KE. (2018). Population genomics of hypervirulent Klebsiella pneumoniae clonal-group 23 reveals early emergence and rapid global dissemination. Nature Communication. 13;9(1):2703. 🔗
- Gamage MA, Liao C, Cheah IR, Chen Y, RX Lim D.R.X., Ku, JWK, Chee RSLC, Gengenbacher M, Seebeck FP, Halliwell B and Gan YH. (2018). The proteobacterial species Burkholderia pseudomallei produces ergothioneine which enhances virulence in mammalian infection. FASEB J. Jun 11:fj201800716. doi: 10.1096/fj.201800716. 🔗
- Lee IR, Sng E, Lee KO, Molton JS, Chan M, Kalimuddin S, Izharuddin E, Lye DC, Archuleta S, Gan YH. (2017). Comparison of diabetic and non-diabetic human leukocytic responses to different capsule types of Klebsiella pneumoniae responsible for causing pyogenic liver abscess. Front Cell Infect Microbiol. 2017 Sep 7;7:401. doi: 10.3389/fcimb.2017.00401. 🔗
- Gamage A, Lee KO, Gan YH. (2017). Anti-cancer drug HMBA acts as an adjuvant during intracellular bacterial infections by inducing Type I IFN through STING. J Immunol. 199(7):2491-2502. 🔗
- Tan YH, Gamage A, Gan YH. (2017). Complement-activated vitronectin enhances the invasion of non-phagocytic cells by bacterial pathogens Burkholderia and Klebsiella. Cell Microbiol. 2017 Aug;19(8). doi: 10.1111/cmi.12732. 🔗
- Lee IR, Molton JS, Wyres KL, Gorrie C, Wong J, Hoh CH, Teo J, Kalimuddin S, Lye DC, Archuleta S, Holt KE and Gan YH(2016). Differential host susceptibility and bacterial virulence factors driving Klebsiella liver abscess in an ethnically diverse population. Sci Rep. 6:29316. 🔗
- Wong J, Chen Y and Gan YH. (2015). Host Cytosolic Glutathione Sensing by a Membrane Histidine Kinase Activates the Type VI Secretion System in an Intracellular Bacterium. Cell Host & Microbe. 18, 1-11. 🔗
- Lim YT, Jobichen C, Wong J, Limmathurotsakul D, Li S, Chen Y, Raida M, Srinivasan N, MacAry PA, Sivaraman J, Gan YH. (2015). Extended loop region of Hcp1 is critical for the assembly and function of type VI secretion system in Burkholderia pseudomallei. Sci Rep. Feb 4; 5:8235. 🔗
- Teh BE, French CT, Chen Y, Chen IG, Wu TH, Sagullo E, Chiou PY, Teitell MA, Miller JF, Gan YH. (2014). Type three secretion system-mediated escape of Burkholderia pseudomallei into the host cytosol is critical for the activation of NFκB. BMC Microbiol.May 6;14:115. 🔗
- Gamage AM, Lee KO, Gan YH. (2014). Effect of oral N-acetyl cysteine supplementation in Type 2 diabetic patients on intracellular glutathione content and innate immune responses to Burkholderia pseudomallei. Microbes Infect. 2014 Aug;16(8):661-71. 🔗
- Chen Y, Schröder I, French CT, Jaroszewicz A, Yee X, Teh BE, Toesca IJ, Miller JF, Gan YH. (2014). Characterization and analysis of the Burkholderia pseudomallei BsaN virulence regulon. BMC Microbiol. Aug 1;14(1):206. 🔗
- Ooi WF, Ong C, Nandi T, Kreisberg JF, Chua HH, Sun G, Chen Y, Mueller C, Conejero L, Eshaghi M, Ang RM, Liu J, Sobral BW, Korbsrisate S, Gan YH, Titball RW, Bancroft GJ, Valade E, Tan P. (2013) The Condition-Dependent Transcriptional Landscape of Burkholderia pseudomallei. PLOS Genetics. 9(9):e1003795. 🔗
- Lee SH, Wong RR, Chin CY, Lim TY, Eng SA, Kong C, Ijap NA, Lau MS, Lim MP, Gan YH, He FL, Tan MW, Nathan S. (2013). Burkholderia pseudomallei suppresses Caenorhabditis elegans immunity by specific degradation of a GATA transcription factor. PNAS. 110(37):15067-72. 🔗
- Gan YH. (2013). Host susceptibility factors to bacterial infections in Type 2 diabetes. PLOS Pathogen. 2013 Dec;9(12):e1003794. 🔗
- Tan KS, Lee KO, Low KC, Gamage AM, Liu Y, Tan GY, Koh HQ, Alonso S and Gan YH. (2012). Glutathione deficiency in Type 2 diabetes impairs cytokine responses and control of intracellular bacteria. J. Clin. Invest. 122(6), 2289-2300. 🔗
- Chen Y, Wong J, Sun GW, Liu Y, Tan GY and Gan YH. (2011). Regulation of Type VI Secretion System during Burkholderia pseudomallei infection. Infect Immun 79(8), 3064-3073. 🔗
- Sun GW and Gan YH. (2010). Unraveling type III secretion systems in the highly versatile Burkholderia pseudomallei. Trends in Microbiology. 18(12), 561-568. 🔗
- Sun GW, Chen Y, Liu Y, Tan GY, Ong C, Tan P and Gan YH. (2010). Identification of a regulatory cascade controlling Type Three Secretion System 3 gene expression in Burkholderia pseudomallei. Mol. Microbiol. 76(3), 677-689. 🔗
- Tan KS, Chen Y, Lim YC, Tan GY, Liu Y, Lim YT, MacAry P and Gan YH. (2010). Suppression of host innate immune response by Burkholderia pseudomallei through the virulence factor TssM. J. Immunol. 184,5160-5171. 🔗
- Lee YH, Chen YH, Ouyang X and Gan YH. (2010). Identification of tomato plant as a novel host model for Burkholderia pseudomallei. BMC Microbiology. 10:28. 🔗
- Breitbach K, Sun GW, Ko¨hler J, Eske K, Wongprompitak P, Tan G, Liu Y, Gan YH and Ivo Steinmetz I. (2009). Caspase 1 mediates resistance in murine melioidosis. Infect. Immun. 77(4), 1589-1595. 🔗
- Ye Z, Lee CML, Sun GW and Gan YH. (2008) Burkholderia pseudomallei infection of T cells leads to T cell costimulation partially provided by flagellin. Infect. Immun. 76, 2541-2550. 🔗
- Hii CS, Sun GW, Goh JWK, Lu J, Stevens MP and Gan YH. (2008) IL-8 induction by Burkholderia pseudomallei can occur without Toll-like receptor signaling but requires a functional Type III secretion system. J. Infect. Dis., 197,1537-1547. 🔗
- Ye ZY and Gan YH. (2007). Flagellin contamination of recombinant heat shock protein 70 is responsible for its activity on T cells. JBC, 282:4479-4484. 🔗
- Koo GC and Gan YH. (2006). The innate interferon gamma response of BALB/c and C57BL/6 mice to in vitro Burkholderia pseudomallei infection. BMC Immunol, 7:19. 🔗
- Gan YH. (2005). Interaction of Burkholderia pseudomallei with host immune response: sleeping with the enemy? J. Infect. Dis., 192:1845-1850. 🔗
- Sun GW, Lu J, Pervaiz S, Cao WP and Gan YH. (2005). Caspase-1 dependent macrophage death induced by Burkholderia pseudomallei. Cell. Microbiol., 7(10), 1447-1458. 🔗
- Chen K, Sun GW, Chua KL, and Gan YH. (2005). Modified Virulence of Antibiotic-induced Burkholderia pseudomallei Filaments. Antimicrobial Agents and Chemotherapy, 49(3), 1002-1009. 🔗
- Chua KL, Chan YY and Gan YH. (2003). Flagella, a virulence determinant in Burkholderia pseudomallei. Infect. Immun. 71, 1622-1629. 🔗
- Gan YH, Chua KL, Chua HH, Liu BP, Hii CS, Chong HL and Tan P (2002). Characterization of B. pseudomallei infection and identification of novel virulence factors using a C. elegans host system. Mol. Microbiol. 44, 1185-1197. 🔗
- Liu BP, Koo GC, Yap EH, Chua KL and Gan YH (2002). A model of differential susceptibility to mucosal infection of Burkholderia pseudomallei. Infect. Immun. 70, 504-511. 🔗